The Experiment
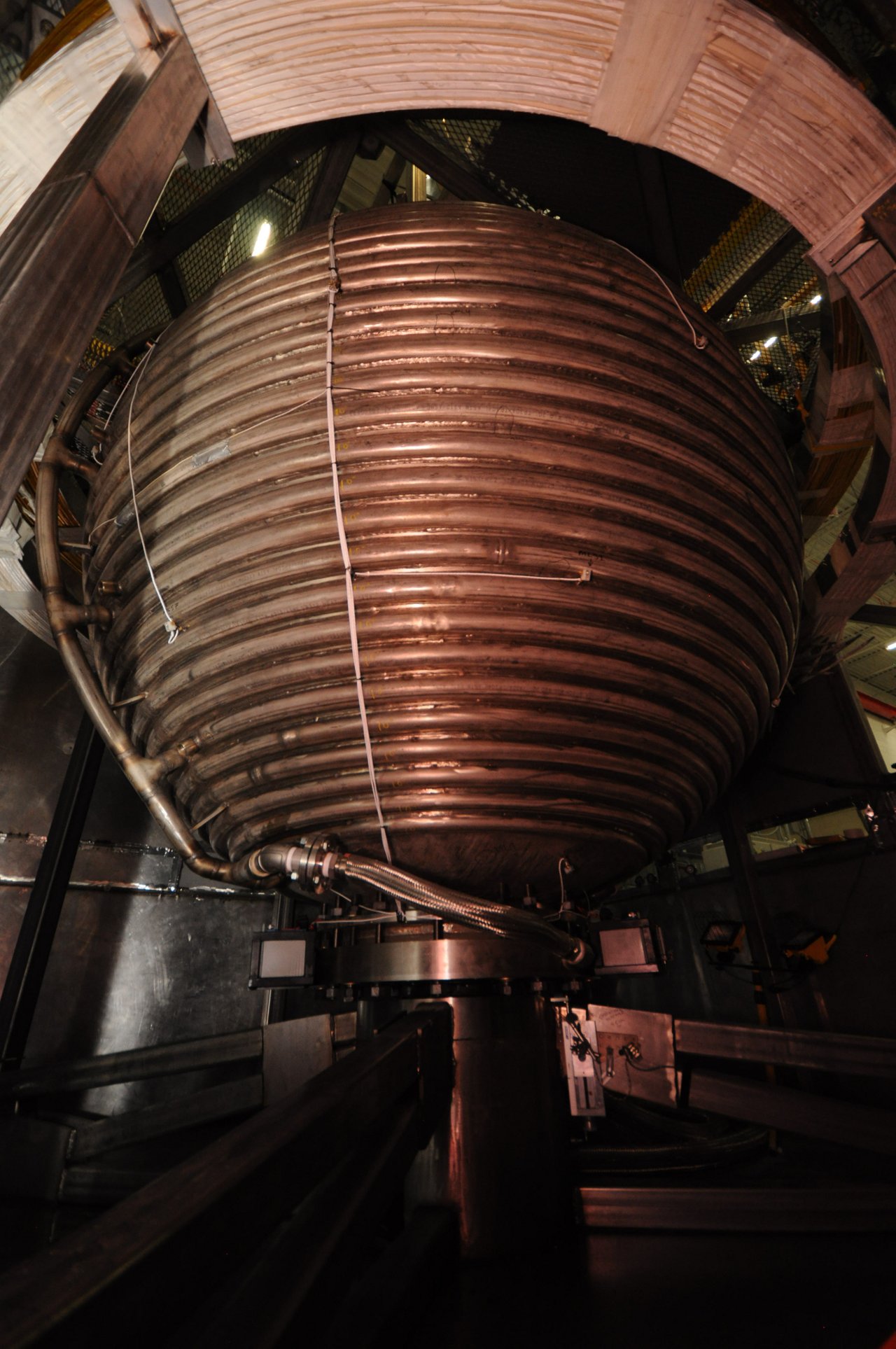
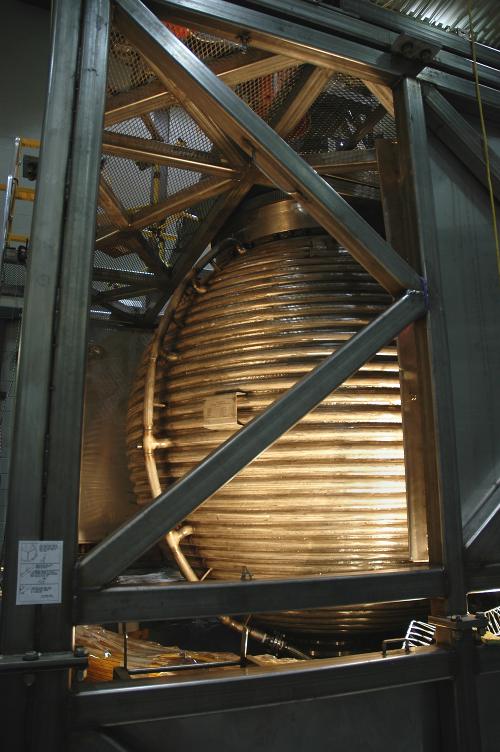
Earth's liquid iron outer core is a self-organized, self-starting electrical generator that provides us with Earth's protective magnetosphere. The core does this with a feedback process that amplifies small magnetic fields into large, self-sustained networks of magnetic field and current flow deep within the Earth.
Like the human-designed electrical generators that generate the bulk of our utility electricity, this "dynamo" process relies on relative motion between complex closed loops of electrically conducting material. Unlike human-designed electrical generators, Earth's core generates electricity and magnetic fields without the benefit of complex winding patterns, ferromagnetic materials, permanent magnets, or electrical insulation. The outer core is a large, continuous mass of liquid metal, mostly iron, and it's much too hot to show any kind of permanent magnetism.
The exact fluid flow conditions allowing an Earthlike dynamo are not well understood. It is thought that the rotation of the Earth is crucial, and it's certain that a fluid with high electrical conductivity is key. The UMD Three Meter experiment is an ambitious experiment funded through a National Science Foundation Major Research Instrumentation grant. Dan Lathrop put together a team including Don Martin, Santiago Andrés Triana, Nolan Ballew, and myself. Together, we designed, constructed, and commissioned the machine that we thought would give the best possible chance to study a self-generating liquid metal dynamo in the laboratory.
Rotating at 2Hz
This experiment is one of the most strongly rotating laboratory experiments on the planet. Though it isn't the fastest spinning experiment, the combination of size and speed means that the Coriolis force is massively dominant over other fluid forces. This organizes the flow into columnar structures and wave motions that make the dynamo process more likely.Engineering
The experiment was a major mechanical, electrical, and thermal engineering project. The outer sphere is a seven-ton stainless-steel oil-heated pressure vessel built by Cincinnati firm Central Fabricators. It has an inch thick wall and rotates on a pair of massive spherical roller bearings bearings up to rotation speeds of four revolutions per second. This three meter diameter spinning tank contains thirteen tons of flammable, highly-reactive liquid sodium metal stirred relative to the outer rotating wall by a one meter diameter inner sphere.
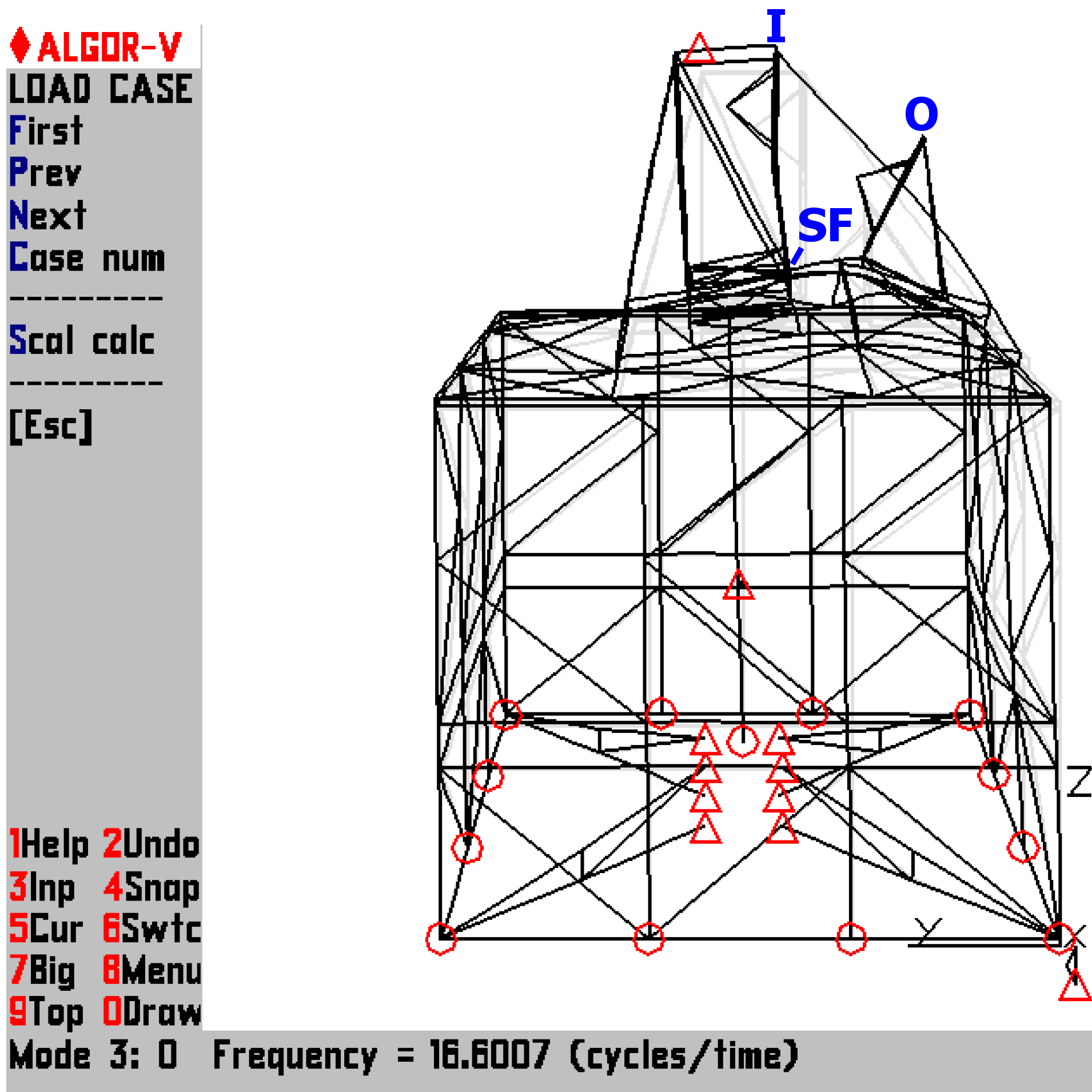
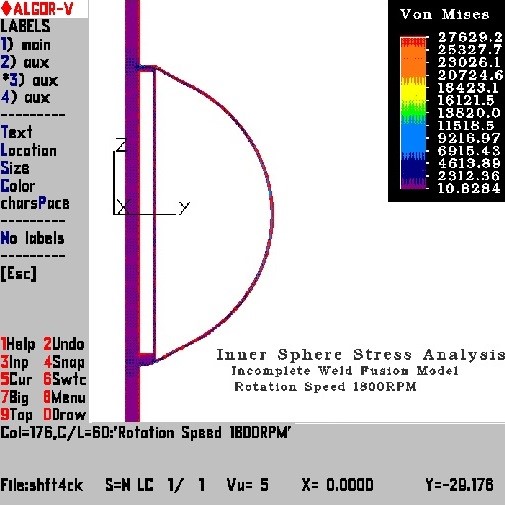
Finite-element analyses of experiment frame (left) and inner sphere (right)
I had a primary role in designing, fabricating, and testing the structural support frame that supports the spinning outer sphere and drive motors. I performed FEA stress analyses and vibrational mode analyses and iteratively improved the frame design to remove resonances in the range of outer sphere rotational speeds. I performed vibrational testing of the completed frame to compare to FEA results and to carefully characterize those frame vibrational modes with frequencies lower than the inner sphere's top rotational speeds. I also used FEA to evaluate the inner sphere's capability to handle centrifugal stresses.
Since outer sphere failures would pose an extreme risk to life safety and university property, verification of the outer sphere's structural design and required FEA analyses were carried out by a PE at a consulting firm specializing in ASME Code pressure vessel design. An outside consulting engineer with decades of experience in rotating equipment design was engaged for overall design review, and university facilities engineers provided guidance, inspections, and design amendments regarding floor loading and building mechanical and electrical modifications.
I designed the reduction drive system for the outer sphere, and worked with engineers at Gates Rubber and Motion Systems to specify the custom-fabricated five foot diameter anodized aluminum timing pulley that drives the outer sphere. I had a major role in designing the hot oil thermal management system that heats and cools the sodium inside the outer sphere. A 120kW circulation heater, five air-cooled radiators, and a 30HP pump recirculate heat transfer oil through the spinning outer sphere's half-pipe oil jacket. I also designed and fabricated heated sodium transfer equipment and gas handling equipment for the lengthy process of filling the outer sphere with sodium.
Instrumentation
I developed and fabricated much of the instrumentation and substantial portions of the control electronics for both the hydrodynamic experiments with water and magnetohydrodynamic experiments with sodium. Several of these projects are highlighted on the electronics section of this site. These included a 24 bit wireless torque sensor on the inner sphere, thermal wall-shear-stress sensors for water experiments, network control and current monitoring for a 80kW magnet power supply, a radio frequency slip ring for ultrasound velocity measurements, a rotating acquisition computer, and numerous other analog signal conditioning systems, scientific sensors, and utility monitoring electronics.
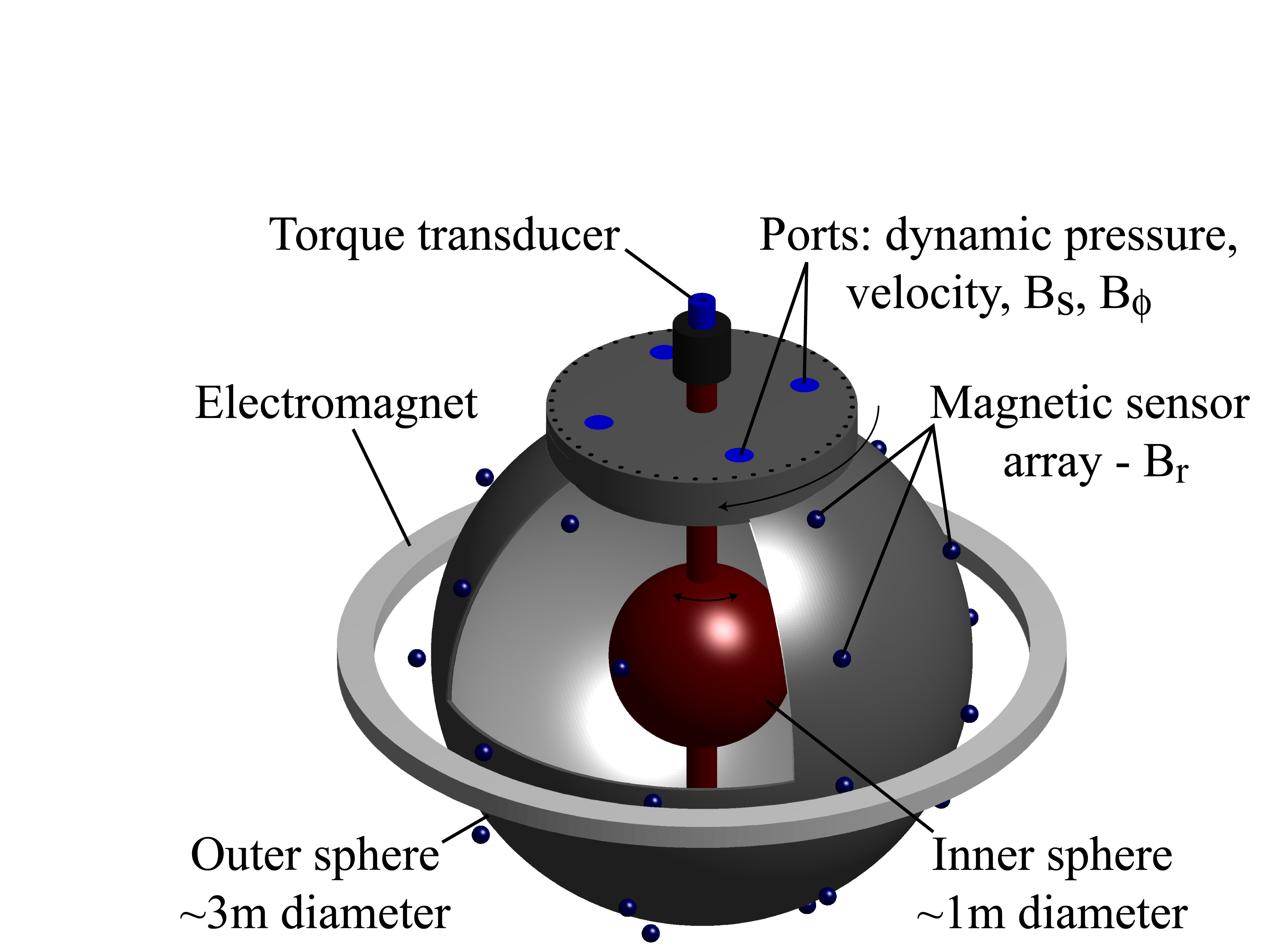
Sensor layout for magnetohydrodynamic experiments.
An array of Hall-effect sensors provide measurements of the spherical-radial component of magnetic field just outside the surface of the outer sphere. Fields induced from an intentionally applied field by the turbulent flow of sodium are a primary measurement in the sodium experiments, allowing a great deal of insight into fluid flow phenomena otherwise obscured by the opaque liquid and seamless wall.
Thirty-one sensors are arranged around the sphere in an optimized pattern developed by Henri-Claude Nataf and Simon Cabanes from ISTerre, who collaborated substantially on the commissioning of sodium experiments. Henri-Claude Nataf remains an active collaborator in the project. We used a vertical linear motion rail and high-resolution angle encoder on the outer sphere to accurately measure the installed position of the Hall sensors in cylindrical coordinates to within a few millimeters. Two more Hall sensors are installed in a tube that penetrates some distance into the sodium to measure the strong azimuthal internal field that is generated from radial and vertical field by differential rotation.
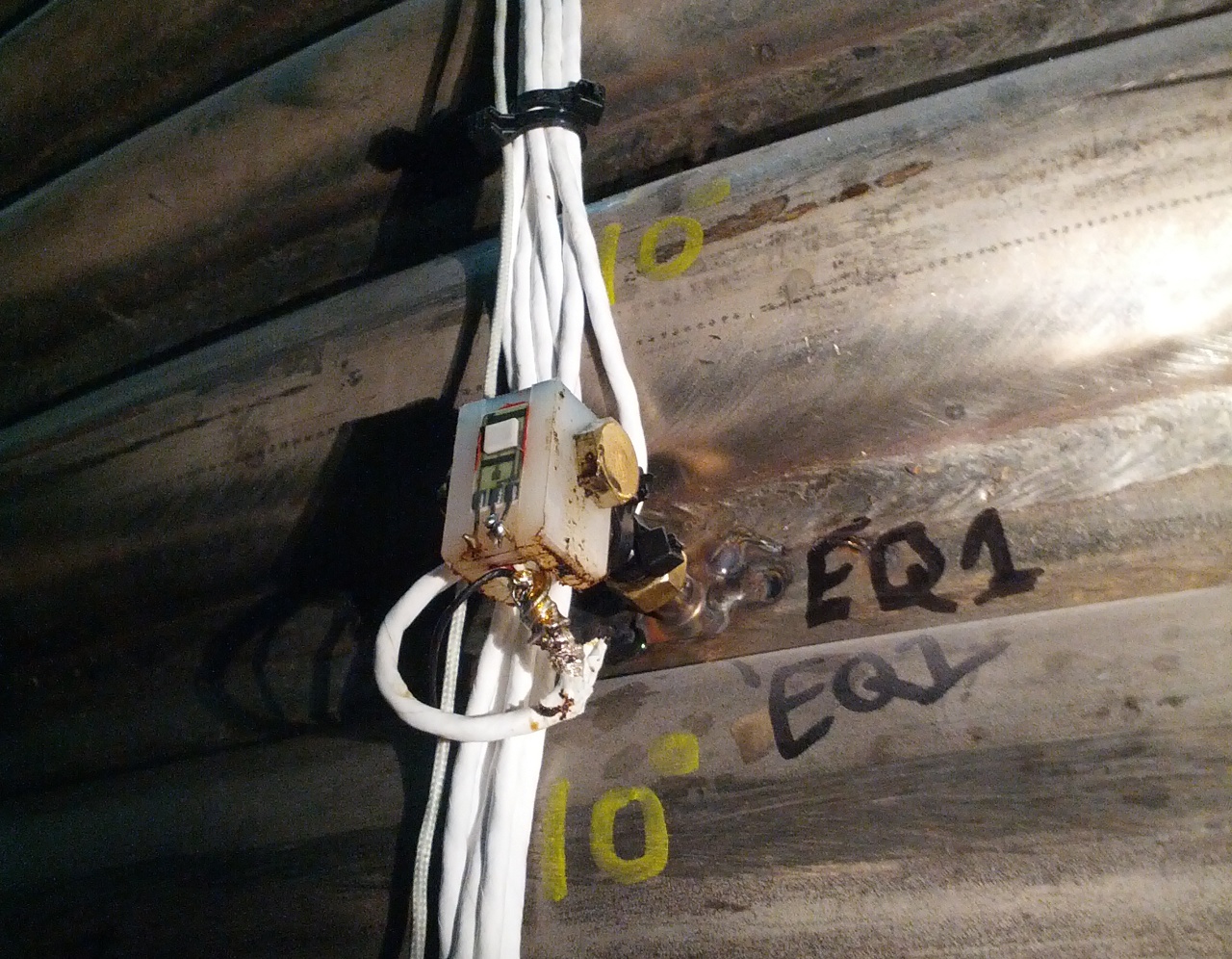
Radial Hall sensor array element.
Results - Torque Scalings
The experiment has not, at the time of this writing, generated a self-sustaining magnetic field as was originally hoped. Since my departure from the project, it has been run up to full speed and power over the entire range of accessible parameters. Substantial amplification of externally applied fields has been observed, but not a self-sustaining liquid metal dynamo. However, a number of highly novel and geophysically relevant scientific results about rotating and/or magnetized flow have come out of the work.
Some of the most interesting results from my work explored the transport of angular momentum between the inner and outer spherical boundaries. Angular momentum is one of the fundamental conserved quantities in rotating flows, and the torque on the rotating boundaries of a rotating volume of fluid provides an integrated measurement of the flux of angular momentum into or out of the fluid at that boundary. Particularly interesting were striking effects of large-scale flow transitions on the boundary torques. The plot below shows the torque scaling as a function of Rossby number, which is the nondimensional ratio of the difference in sphere rotation and the overall rotation rate.
Non-dimensional torque vs. Rossby number
Results - Turbulent to Turbulent Transitions
One of the most surprising findings were intermittent transitions between two extremely different flow states co-existing at fixed Rossby number. Here, with the inner and outer spheres spinning at fixed, tightly controlled rates for hours at a time, the flow spontaneously transitions between a low-torque high-speed vortex and a high-torque heavily-mixed breakdown state. The correlated transitions in azimuthal velocity measured using ultrasound velocimetry and inner sphere torque are shown below.
Non-dimensional torque G and azimuthal velocity uφ at the L-H turbulent state transition.
The movies below show the vast difference between the two flow states co-existing at the same Rossby number. Again, there are no changes in inner or outer sphere rotation rates to trigger the transitions. The high-torque breakdown is spontaneous. The movie is taken looking into one of the instrumentation ports on the experiment schematic further up this page. The movie on the left below was taken as soon as we noticed the torque spike at one of the L->H transitions in the figure above. The average flow has nearly ceased, leaving large fluctuations in all directions, despite the inner sphere surface below moving from right to left, just as rapidly as it is in the right-hand movie.
Dye injection in high (left) and low-torque (right) flows coexisting at the same parameters.
Check out my publications page for more results from my geophysics work with the 3m experiment and other liquid metal and rotating experiments that I've been involved with. In addition to links to papers, I've hosted some selected conference posters and slide decks on this site for easy browsing.
TL;DR
For more on the 3m experiment and more background on the scientific goals of laboratory dynamo experiments, or if you just need a video break, let Derek from Veritasium and my former Ph.D. advisor give you a quick tour of the subject: